Translate this page into:
Dosimetric analysis of LAD dose in left-sided breast cancer radiotherapy with deep inspiratory breath hold
*Corresponding author: Vrushab Rao, Department of Cyberknife Radiosurgery and Radiation Oncology, Ruby Hall Clinic, Pune, India. raovrushab@gmail.com
-
Received: ,
Accepted: ,
How to cite this article: Rao V, Munshi M, Vatyam SNK, Moundekar P, Kurup S, Mane A, et al. Dosimetric analysis of LAD dose in left-sided breast cancer radiotherapy with deep inspiratory breath hold. Indian J Breast Imaging. 2024;2:5-12. doi: 10.25259/IJBI_12_2023
Abstract
Objectives
To analyze the dose to the left anterior descending artery (LAD) in patients who have received radiotherapy for left breast cancer with Deep Inspiratory Breath Hold (DIBH) technique and compare it with other cardiac dosimetric parameters, as well as the accepted dose constraints.
Materials and Methods
20 patients (10 prospective and 10 retrospective) were selected for this study. All patients underwent 2 non-contrast radiation planning CT scans of 2.5 mm thickness - one with DIBH and one with free breathing. Contouring was done using the Radiation Therapy Oncology Group (RTOG) guidelines. LAD was delineated and given a PRV of 3 mm and 5 mm. Dose-volume histograms (DVH) were used to obtain the data from the approved plans.
Results
The lung volume receiving 17 Gy in percentage, Dmean of the heart, LAD Dmean and Dmax, and the Dmean and Dmax received by 3 mm and 5 mm PRVs were both very well achieved when compared to the dose constraints given by the DBCG HYPO trial. The study found a higher correlation between the mean heart dose and the 5 mm PRV dose (R2 = 0.81 and 0.71 respectively for the mean and max dose) than the 3 mm PRV, and a positive correlation between the heart dose and LAD making it a useful structure for predicting acute cardiac events.
Conclusion
The study of 20 patients found that DIBH is effective to minimize cardiac dose and potentially cardiac toxicity, with heart and LAD doses being comparable or lower compared to other studies. The LAD doses recorded were significantly less than those in non-DIBH studies, demonstrating the feasibility of routine contouring and recording LAD dose in left-sided breast radiation patients. Further research is needed to determine the dosimetry and clinical consequences of the Dmean and Dmax of the 5mm PRV to the LAD.
Keywords
Left anterior descending coronary artery
Deep inspiratory breath hold
Left-sided breast cancer
Radiotherapy
INTRODUCTION
Radiation therapy (RT) is an integral part in the treatment of breast cancer, and nearly 75% of the patients post breast conservation surgery and 29.5% of patients post-mastectomy will require radiotherapy as a part of their treatment.[1] It eliminates subclinical disease following surgical excision of the overtly visible tumor. Radiation also lowers local recurrence rates and improves breast cancer–specific survival and overall survival by 20% in patients with early stage breast cancer who have undergone breast-conserving surgery, as well as in node-positive patients who have had a mastectomy. The Early Breast Cancer Trialists’ Collaborative Group (EBCTCG) showed conclusively that radiotherapy lowered the chances of any recurrence by half and the breast cancer–related mortality by about one-sixth, cementing its role as an integral part of early and locally advanced diseases.[2]
Radiotherapy planning for breast cancer is usually done using tangential beams to avoid direct entry of radiation beams into the lungs and heart. The technique of planning has evolved from 2D planning to 3DCRT, and to Forward Planned Intensity Modulated Radiotherapy (IMRT), which is currently the standard of care. The addition of subfields aids in achieving better dose homogeneity while protecting the vital organs at risk, namely, heart for left-sided breast cancers and lungs. Minimizing the radiation dose received by the heart has been a key component of radiation dose planning, especially in left-sided breast cancer cases. This is especially important as patients often receive anthracyclines or trastuzumab as a part of treatment – both of which are known to be cardiotoxic.[3] Hypertension and diabetes can further this risk and is common amongst our population. Darby et al. showed that there is a 7.4% increment in risk of ischemic heart disease per gray of radiation to the heart and the effects were seen between 5 and 30 years.[4]
Traditionally, mean heart dose has been used to calculate dose constraints for heart; however, it has been found that the dose received by the left anterior descending (LAD) artery correlated with adverse cardiac events.[5] This warrants prospective contouring and planning to limit the dose received by the LAD.
Deep Inspiratory Breath Hold (DIBH) is the most widely used technique to reduce cardiac doses, as it allows and aids physical separation of the chest wall or the overlying breast tissue from the heart. Significant reduction in cardiac doses due to DIBH have been shown by various studies.[6–8] Uncertainties in doses to a structure such as LAD exist due to cardiac motion which needs to be accounted for. Planning organ at risk volume (PRV) is a convenient method to account for motion of such small structures.
This study analyzes the dose of the LAD in patients who have received radiotherapy for left-sided breast cancer with DIBH technique and compares it with other cardiac dosimetric parameters of the patients, as well as the accepted dose constraints.
MATERIALS AND METHODS
Twenty cases (10 prospective and 10 retrospective) were selected for this study from a single institute. The median age of the cohort was 49 years. Only cases who received radiotherapy using the UK START-B regimen, [9] that is, 40 Gy in 15 fractions and planned using forward IMRT after breast conservation surgery (BCS) or mastectomy were selected. Eighteen patients who had undergone BCS received a boost to a dose of 12.5 Gy in five fractions using mini-tangents. Cases where the LAD was not visualized despite changing the exposure were excluded. All patients underwent two non-contrast radiation planning CT scans of 2.5 mm thickness – one with DIBH and one with free breathing on a GE Discovery CT 590 RT with RGSC. The optimal settings were found to be 120 kV and 340 mA. After patient coaching and assessment of the patient’s breathing pattern, the amplitude of breath-hold was captured by an infrared sensor camera and corresponded to the DIBH CT taken simultaneously. The free-breathing scan was taken subsequently.
The RT Oncology Group breast cancer atlas was referred to define the clinical target volume (CTV). All ipsilateral breast tissue, except the muscles underneath and the overlying skin, was included in the CTV. The posterior boundary of the CTV in a chest wall radiation case stopped at the anterior pleural surface, which included the ribs and intercostal muscles. Internal mammary chain or axilla is not included routinely for node positive patients in our practice. With revisions made to the medial, lateral, inferior, and superior boundaries, the planning target volume (PTV) margin was 3 mm. The organs at risk that were marked included both lungs, the heart, LAD with a 3 mm and 5 mm PRV, the spinal cord with a 3 mm PRV, and esophagus. The LAD was contoured from its origin near the left coronary artery continuing below the right pulmonary vessel, and coursing along the anterior interventricular groove till the cardiac apex [Figure 1]. In three cases, a stent in situ aided the contouring. Planning was done with forward IMRT on the EclipseTM planning system version 16.1 by Varian Medical Systems, with subfields added to achieve better dose distribution [Figure 2]. The volume receiving 97% of the dose was planned to be > 95% of the PTV for every plan. Dose constraints were set based on the Danish breast cancer group (DBCG) HYPO trial.[10]
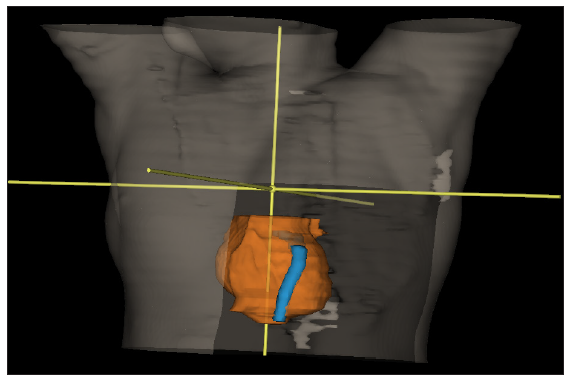
- Three millimeter Left anterior descending artery (LAD) Planning Organ-at-risk volume (PRV) and heart contour.
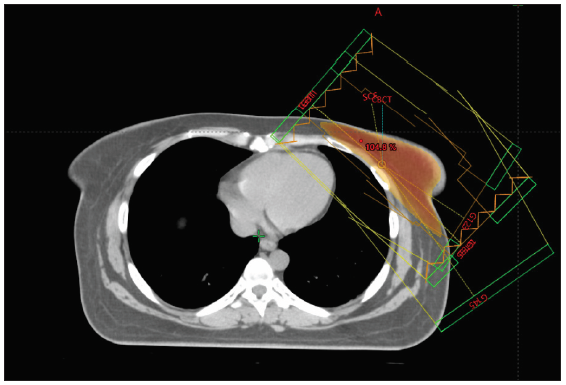
- External beam planning.
Dose-volume histograms (DVH) were used to obtain data from the approved plans. The parameters derived from the DVH were as follows – volume of the lung receiving 17 Gy in percentage, mean dose of the heart, volume of the heart receiving 17 Gy and 35 Gy, mean and max doses received by the LAD, and the mean and max doses received by the 3 mm and 5 mm PRV given to the LAD. The data were then tabulated for statistical analysis. All patients were treated with Varian TruebeamTM STx with daily kVCBCT image guidance and 6D couch correction.
Statistical Analysis
Each data set was tested for normal distribution using the Kolmogorov–Smirnov Test. Correlation between various parameters was done using the Pearson’s correlation coefficient. The comparison of our data with the existing guidelines was done using the unpaired t-test. The statistical tests were done using JASP 0.17.3. We compared the doses from our study with those reported from other similar studies.
RESULTS
There were 18 patients with BCS and 2 patients who had undergone mastectomy. Thirteen patients were node positive and received radiation to the supraclavicular fossa, while seven patients were node negative. The doses achieved in our cohort of patients are listed in Table 1.
Constraints | Mean observed value |
---|---|
Vol of Left Lung receiving 17 Gy | 14.289 ± 4.688 (%) |
Vol of Heart receiving 17 Gy | 1.4759% |
Vol of Heart receiving 35 Gy | 0.4565% |
Mean dose Heart | 1.784 Gy ± 1.15 Gy |
Mean dose LAD | 6.079 Gy ± 5.709 Gy |
Max dose LAD | 18.4835 Gy ± 14.047 Gy |
Mean dose PRV 3 mm | 6.046 Gy ± 5.149 Gy |
Max dose PRV 3 mm | 24.602 Gy ± 13.232 Gy |
Mean dose PRV 5 mm | 6.1535 Gy ± 4.9546 Gy |
Max dose PRV 5 mm | 28.1565 Gy ± 12.419 Gy |
Gy: Gray; PRV: Planning organ-at-risk volume; LAD: Left anterior descending artery.
When compared to the dose constraints given by the DBCG HYPO trial, the volume of the heart receiving 17 Gy and 35 Gy, and the volume of the left lung receiving 17 Gy were very well achieved. The Dmax of the LAD was comparable to the expected value. We found that the dose received by the 5 mm (mean and max) PRV had a higher correlation with the mean heart dose than the 3 mm PRV (R2 = 0.81 and 0.71, respectively, for the mean and max dose) [Figures 3, 4, and 5]. There was a positive correlation between the mean dose of the heart and LAD (R2 = 0.786), which can be used as a more accurate structure to predict acute cardiac events.
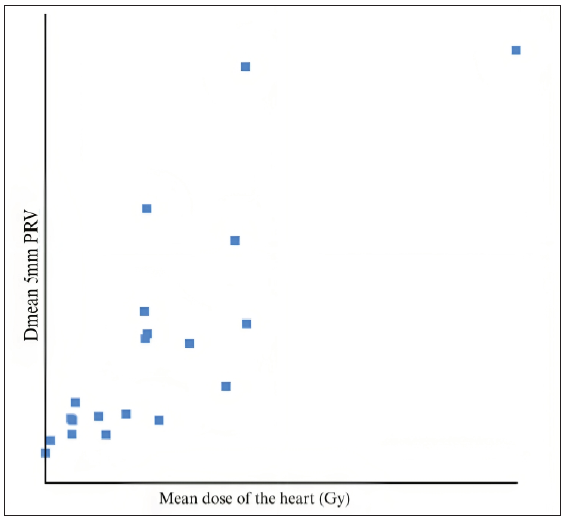
- Mean dose of the heart vs. Dmean of 5 mm PRV. As this is a graph of correlation between the PRV and the mean dose, with each point representing a patient/value.
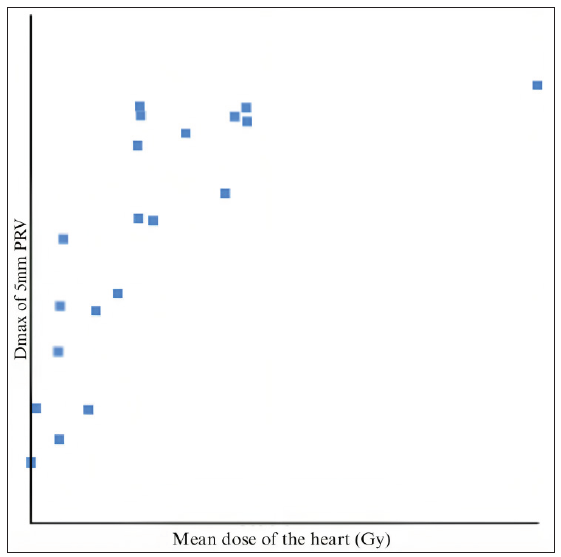
- Mean dose of the heart vs. Dmax of 5 mm PRV. PRV: Planning Organ at Risk Volume.
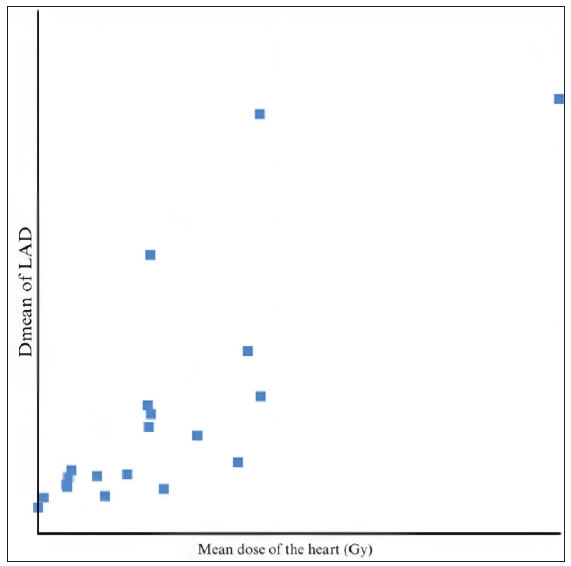
- Mean dose of the heart vs. Dmean of LAD. LAD: Left Anterior Descending Artery.
DISCUSSION
Radiotherapy has been proven to be a vital tool in the arsenal of oncologists to treat breast cancers in the postoperative setting. Yang and Ho have summarized the role of RT in breast cancers. After the surgical excision of the overtly obvious tumor, radiation treatment plays a crucial part in the care of breast cancer by curing the subclinical illness. In patients with early stage breast cancer following breast-conserving surgery as well as in node-positive patients who have had a mastectomy, radiation decreases local recurrence rates and improves breast cancer–specific survival.[11] The Early Breast Cancer Trialists’ Collaborative Group (EBCTCG) studied the effect of radiotherapy after breast-conserving surgery on 10-year recurrence and 15-year breast cancer death and reported that breast cancer death rates are reduced by roughly a sixth, and the rate of disease recurrence is cut in half in the preserved breast after radiation. While the proportional benefits had little variation between different groups of women, the absolute benefit varied significantly between different patient characteristics.[2] The benefit of RT was confirmed by other trials as well.[12–16] The current guidelines by National Comprehensive Cancer Network (NCCN) state that RT must be given in all cases who have undergone BCS, and in mastectomies with large tumors or node-positive cases.
Exposure to radiation dose has been documented as a key risk factor in the development of various heart diseases. Darby et al. concluded that the probability of developing ischemic heart disease increased by 7.4% for every gray of radiation received by the heart. The effects were seen from as early as 5 years post breast radiotherapy till three decades post RT as well.[4] There, however, was no apparent threshold with respect to the dose that was detected. According to the Surveillance, Epidemiology and End Results database (SEER), radiation treatment for left-sided breast cancer prior to 1990 was associated with a higher relative mortality, particularly in younger patients and those who also had chemotherapy like anthracyclines or trastuzumab.[17] The pathophysiology of radiation-related cardiotoxicity is micro- and macroangiopathy in the coronary arteries, which may lead to myocardial fibrosis and coronary artery disease.[18]
The UK START trial B results indicate that a radiation treatment plan that delivers 40 Gy in 15 fractions appears to have local-regional tumor recurrence and late adverse effect rates that are comparable to the conventional treatment fractionation of 50 Gy in 25 fractions.[9] Piroth et al. have described the possible mechanisms of cardiotoxicity by radiotherapy and have also stated the DEGRO breast cancer expert panel recommendations for dose constraints that are listed in Tables 2 and 3, respectively.[19]
Pathophysiological findings |
1. RT leads to inflammation, oxidative effects, cytokine activity, and endothelial damage, and leads to an accelerated atherosclerotic process. 2. Radiation dosages of less than 2 Gy can cause arteriosclerosis because they cause inflammation in endothelial cells, which are susceptible to radiation. |
Angiographic findings |
1. There was an increase in clinically significant coronary artery stenoses in the predefined hotspot areas in patients who underwent left-sided WBI/chest wall RT compared to patients who did not receive RT in these areas. 2. A four- to seven-fold risk increase was seen in significant stenosis for the mid and distal LAD in radiation hotspot areas. 3. The median dose to the damaged vs. undamaged coronary segments was 30.3 vs. 26.3 Gy in mediastinal Hodgkin’s lymphoma cases that received RT. |
Functional imaging |
1. New perfusion defects were detected in 27, 29, 38, and 42% of patients after 6, 12, 18, and 24 months, respectively. 2. Perfusion defects were seen in 10–20% of patients if the left ventricle was included in the radiation portals, as opposed to 50%–60% if <5% versus ≥5% of the left ventricle was included. |
Strain rate imaging |
1. A strain and strain-rate reduction could be seen post RT. 2. A considerable reduction in strain, indicated as a reduction in systolic myocardial deformation, could be seen in the apical segments receiving >3 Gy vs. 3 Gy. |
Serum biomarkers |
1. Cardiac biomarkers such as N-terminal pro-B-type natriuretic peptide (NT-proBNP) or troponin (TnI) increase after left-sided breast RT. 2. Patients with higher high-sensitivity cardiac troponin T (hscTnT) had a higher mean LAD dose and a higher V15, V20, and V30 for the LAD. |
RT: Radiation therapy; WBI: Whole breast irradiation; Gy: Gray; LAD: Left anterior descending artery.
Structure | Constraints |
---|---|
Dmean Heart | <2.5 Gy |
DmeanLV | <3 Gy |
V5LV | <17% |
V23LV | <5% |
DmeanLAD | <10 Gy |
V30LAD | <2% |
V40LAD | <1% |
DEGRO: German Society of Radiation Oncology; LV: Left ventricle; LAD: Left anterior descending artery.
The Danish Breast Cancer Group (DBCG) HYPO Trial compared hypofractionated with standard fractionated radiotherapy in early breast cancers and DCIS. The trial found that the 9-year locoregional recurrence risk was low, and both arms were comparable in terms of toxicity. In addition, the study provided stricter guidelines for dose constraints for the Organs at risk (OARs) that are enlisted in Table 4.[5]
CTVp | |
---|---|
V95% | ≥95% |
V105%–V107% | ≤2% |
V107%–V110% | <2cm3 |
Dmax | ≤110% |
Lung | |
V17 Gy | ≤25% |
Heart | |
V17 Gy | ≤10% |
V35 Gy | ≤5% |
LADCA | |
Dmax | ≤17 Gy |
DBCG: Danish Breast Cancer Cancer Group; LAD: Left anterior descending artery; Gy: Gray; PRV: Planning organ-at-risk volume. CTVp: Clinical Target Volume of the primary tumor; LADCA: Left Anterior Descending Coronary Artery.
Yeung et al. showed that when patients received breast or chest wall radiation with regional nodal irradiation, including internal mammary chain nodes, DIBH permitted a higher decrease in mean heart and LAD doses than when patients received whole breast radiotherapy (WBRT) without breath hold.[6] Wennstig et al. was one of the first to deduce that the LAD was a surrogate indicator of cardiac events. Radiation exposures to the LAD in women receiving conventional 3DCRT for breast cancer between 1992 and 2012 remained high and were linked to a higher need for coronary intervention in the middle of the LAD.[20] The results of the trial by Zureick et al. showed that any cardiac event, whether significant cardiac event or mild, was related with higher LAD Dmean, LAD Dmax, and heart Dmean values. A threshold LAD Dmean EQD2 of 2.8 Gy was identified.[5] Duma et al. suggested that the dose to the LAD or the Dmean to the left ventricle were not surrogate parameters for the Dmean heart in every case. Hence, a heart Dmean is non-specific and contouring of the different heart substructures is needed to ascertain late toxicities.[21] Data from lung radiotherapy have also found that mean heart dose (MHD) does not correlate with major adverse cardiovascular events (MACE) and LAD is a better surrogate marker V15 Gy.[22] Jacob et al., illustrated that MHD is insufficient to reliably predict each patient’s dosage to the LV and coronary arteries, especially the LAD, and that distribution of doses to individual cardiac substructures may be necessary to accurately study radiation-induced cardiotoxicity. The proportion of the variance in the Dmean of the substructure that was predictable from MHD was moderate to low, especially LAD.[23] Sakyanun et al. showed that radiation doses to the LAD area, heart, and lungs are significantly decreased when the DIBH method is used to treat left breast cancer. The study also proved a positive correlation of the mean heart dose with the breast size in cubic centimeter and the maximum heart distance measured in centimeter.[8]
Delineation of LAD can be problematic, compounded by its physiological motion. Studies have suggested a 1 cm margin to account for this.[24] In our study, we chose to make two PRVs, 3 mm and 5 mm, to account for this motion.[25–27] Knöchelmann et al. showed a positive linear correlation between LAD Dmean and MHD; however, the mean dose of the ipsilateral lung (MLD) was significantly higher in DIBH compared to free breathing scans. They also found that ipsilateral lung volumes receiving at least 5 Gy, 10 Gy, and 15 Gy (V5, V10, V15) were significantly higher in DIBH compared to nDIBH in both fractionation schemes.[28]
Garg and Kumar studied doses to the heart and LAD in left-sided post mastectomy radiotherapy planned with 3DCRT and inverse planning IMRT with 5–7 mostly tangential beams. They noted that 3DCRT technique had significantly lower doses to the heart and LAD than IMRT plans. They also found that doses to LAD were significantly higher than mean heart dose in both 3DCRT and IMRT plans.[29] In a dosimetric study by Ahmad et al., 3DCRT, VMAT, and IMRT plans were compared for ultra-hypofractionated left-sided breast radiotherapy, without DIBH. Unsurprisingly, they found that although coverage and V25 OAR doses were better with VMAT, the low doses of V5 were of concern.[30]
The comparison of our data with a few similar studies is enlisted in Table 5.
Study | Free Breathing ± DIBH studied | Fractionation Schedule | Mean dose Heart in Gy (Free breathing/DIBH) | Mean dose LAD in Gy (Free breathing/DIBH) | Max dose LAD in Gy (Free breathing/DIBH) | Mean dose PRV 5 mm in Gy (Free breathing/DIBH) | Max dose PRV 5 mm in Gy (Free breathing/DIBH) |
---|---|---|---|---|---|---|---|
Mendez et al.[31] | Free breathing | 50 Gy/25# | 2.3 | >28 | |||
Poitevin-Chacón et al.[32] | Free breathing | 50 Gy/25# | 3.7 | 24.48 | 33.205 | ||
Hayden et al.[27] | Free breathing + DIBH | 50 Gy/25# | 6.9/3.9 | 31.7/21.9 | 51.6/45.6 | ||
Gaál et al.[33] | Free breathing + DIBH | 50 Gy/25# | 3.16/1.38 | 13.05/4.55 | 39.81/18.92 | ||
Gaál et al.[33] | Free breathing + DIBH | 50 Gy/25# | 4.87/2.13 | 18.95/7.47 | 47.03/31.86 | ||
Sakyanun et al.[8] | Free breathing + DIBH | 50 Gy/25# | 19.84/11.48 | 5.38/2.95 | |||
Naimi et al.[34] | Free breathing | 40 Gy/15# | 3.08 | 11.45 | 29.5 | ||
Bartlett et al.[35] | Free breathing + DIBH | 40 Gy/15# | 0.66/0.44 | 7.8/2.9 | 36.8/21 | ||
Berg et al.[36] | Free breathing + DIBH | 40 Gy/15# | 1.5/1.2 | 11.8/11 | |||
Our study | 40 Gy/15# | 1.784 | 6.079 | 18.483 | 6.153 | 28.1565 |
DIBH: Deep Inspiratory Breath Hold; LAD: Left anterior descending artery; Gy: Gray; PRV: Planning organ-at-risk volume.
STRENGTHS
There are only a few studies that have studied the dosimetry of LAD using the UK START B hypofractionated dose regimen, more so using the DIBH technique. Only selected publications have studied the correlation of PRV-LAD and heart doses.
LIMITATIONS
Our sample size is small, and an accurate comparison of our data to other studies could not be done due to variations in either the fractionation protocols or the planning techniques used. Cardiac toxicity is a long-term complication which requires a long follow-up and clinical correlation.
CONCLUSION
In our cohort of 20 patients, the heart and LAD doses were either comparable or lower when compared to other similar studies of comparable dose fractionation protocols. The LAD doses that we have recorded are significantly less than those reported in the non-DIBH studies. This objectively demonstrates that DIBH is an effective technique to minimize cardiac toxicity. Though challenging in some cases, it is feasible to routinely contour and record LAD dose in patients receiving left-sided breast radiation. Dosimetry and clinical implications of the Dmean and Dmax of the 5 mm PRV to the LAD may be useful in the future, and it requires further research and clinical correlation.
Ethical approval
Since this a dosimetric study and does not involve any active intervention on any patient, the IRB and IEC approval does not apply to this study.
Declaration of patient consent
The authors certify that they have obtained all appropriate patient consent.
Financial support and sponsorship
Nil.
Conflicts of interest
There are no conflicts of interest.
Use of artificial intelligence (AI)-assisted technology for manuscript preparation
The authors confirm that there was no use of artificial intelligence (AI)-assisted technology for assisting in the writing or editing of the manuscript and no images were manipulated using AI.
References
- Defining the need for breast cancer radiotherapy in the general population: A criterion-based benchmarking approach. Clin Oncol (R Coll Radiol). 2007;19:481-9.
- [Google Scholar]
- Effect of radiotherapy after breast-conserving surgery on 10-year recurrence and 15-year breast cancer death: Meta-analysis of individual patient data for 10,801 women in 17 randomised trials. Lancet. 2011;378:1707-16.
- [Google Scholar]
- Anthracycline and trastuzumab-induced cardiotoxicity in breast cancer. Eur Rev Med Pharmacol Sci. 2018;22:2175-85.
- [Google Scholar]
- Risk of ischemic heart disease in women after radiotherapy for breast cancer. N Engl J Med. 2013;368:987-98.
- [Google Scholar]
- Dose to the left anterior descending artery correlates with cardiac events after irradiation for breast cancer. Int J Radiat Oncol Biol Phys. 2022;114:130-39.
- [Google Scholar]
- Cardiac dose reduction with deep inspiration breath hold for left-sided breast cancer radiotherapy patients with and without regional nodal irradiation. Radiat Oncol. 2015;10:200.
- [Google Scholar]
- Cardiac dose reduction using deep inspiratory breath hold (DIBH) in radiation treatment of left sided breast cancer patients with breast conservation surgery and modified radical mastectomy. J Med Imaging Radiat Sci. 2021;52:57-67.
- [Google Scholar]
- The effect of deep inspiration breath-hold technique on left anterior descending coronary artery and heart dose in left breast irradiation. Radiat Oncol J. 2020;38:181-8.
- [Google Scholar]
- The UK Standardisation of Breast Radiotherapy (START) Trial B of radiotherapy hypofractionation for treatment of early breast cancer: A randomised trial. Lancet. 2008;371:1098-107.
- [Google Scholar]
- Dose constraints for whole breast radiation therapy based on the quality assessment of treatment plans in the randomised Danish breast cancer group (DBCG) HYPO trial. Clin Transl Radiat Oncol. 2021;28:118-23.
- [Google Scholar]
- Radiation therapy in the management of breast cancer. Surg Clin North Am. 2013;93:455-71.
- [Google Scholar]
- Role of radiotherapy in the management of breast cancer. J Natl Med Assoc. 1977;69:787-92.
- [Google Scholar]
- The Changing Role of Radiation Therapy in Breast Cancer. In: McGuire W.L., ed. Current Approaches to Therapy. Boston, MA: Springer; 1977.
- [Google Scholar]
- Role of radiotherapy in early breast cancer: An overview. Int J Health Sci (Qassim). 2007;1:259-64.
- [Google Scholar]
- Cardiac mortality after radiotherapy, chemotherapy and endocrine therapy for breast cancer: Cohort study of 2 million women from 57 cancer registries in 22 countries. Int J Cancer. 2020;147:1437-49.
- [Google Scholar]
- Dosimetry of the left anterior descending coronary artery in left breast cancer patients treated with postoperative external radiotherapy. Rep Pract Oncol Radiother. 2018;23:91-6.
- [Google Scholar]
- Heart toxicity from breast cancer radiotherapy: Current findings, assessment, and prevention. Strahlenther Onkol. 2019;195:1-12.
- [Google Scholar]
- The relationship between radiation doses to coronary arteries and location of coronary stenosis requiring intervention in breast cancer survivors. Radiat Oncol. 2019;14:40.
- [Google Scholar]
- Tangential field radiotherapy for breast cancer-the dose to the heart and heart subvolumes: What structures must be contoured in future clinical trials? Front Oncol. 2017;7:130.
- [Google Scholar]
- Mean heart dose is an inadequate surrogate for left anterior descending coronary artery dose and the risk of major adverse cardiac events in lung cancer radiation therapy. Int J Radiat Oncol Biol Phys. 2021;110:1473-79.
- [Google Scholar]
- Is mean heart dose a relevant surrogate parameter of left ventricle and coronary arteries exposure during breast cancer radiotherapy: a dosimetric evaluation based on individually-determined radiation dose (BACCARAT study) Radiat Oncol. 2019;14:29.
- [Google Scholar]
- Safety Margins for the delineation of the left anterior descending artery in patients treated for breast cancer. Int J Radiat Oncol Biol Phys. 2021;109:267-72.
- [Google Scholar]
- Reducing cardiac doses: a novel multi-leaf collimator modification technique to reduce left anterior descending coronary artery dose in patients with left-sided breast cancer. J Med Radiat Sci. 2017;64:114-9.
- [Google Scholar]
- Cardiac dose reduction with deep-inspiratory breath hold technique of radiotherapy for left-sided breast cancer. J Med Phys. 2017;42:123-7.
- [Google Scholar]
- Deep inspiration breath hold technique reduces heart dose from radiotherapy for left-sided breast cancer. J Med Imaging Radiat Oncol. 2012;56:464-72.
- [Google Scholar]
- Left-sided breast cancer irradiation with deep inspiration breath-hold: Changes in heart and lung dose in two periods. In Vivo. 2022;36:314-24.
- [Google Scholar]
- Dosimetric Comparison of the Heart and left anterior descending artery in patients with left breast cancer treated with three-dimensional conformal and intensity-modulated radiotherapy. Cureus. 2022;14:e21108.
- [Google Scholar]
- Dosimetric study comparing 3d conformal radiotherapy (3D-CRT), intensity modulated radiotherapy (IMRT) and volumetric modulated arc therapy (VMAT) in hypofractionated one-week radiotherapy regimen in breast cancer. Cureus. 2022;14:e31860.
- [Google Scholar]
- Evaluation of a new predictor of heart and left anterior descending artery dose in patients treated with adjuvant radiotherapy to the left breast. Radiat Oncol. 2018;13:124.
- [Google Scholar]
- Dosimetry of the left anterior descending coronary artery in left breast cancer patients treated with postoperative external radiotherapy. Rep Pract Oncol Radiother. 2018;23:91-6.
- [Google Scholar]
- Deep-inspirational breath-hold (DIBH) technique in left-sided breast cancer: Various aspects of clinical utility. Radiat Oncol. 2021;16:89.
- [Google Scholar]
- Cardiac substructures exposure in left-sided breast cancer radiotherapy: Is the mean heart dose a reliable predictor of cardiac toxicity? Cancer Radiother. 2021;25:229-36.
- [Google Scholar]
- The UK HeartSpare Study (Stage IB): Randomised comparison of a voluntary breath-hold technique and prone radiotherapy after breast conserving surgery. Radiother Oncol. 2015;114:66-72.
- [Google Scholar]
- The potential benefits from respiratory gating for breast cancer patients regarding target coverage and dose to organs at risk when applying strict dose limits to the heart: Results from the DBCG HYPO trial. Acta Oncol. 2018;57:113-9.
- [Google Scholar]